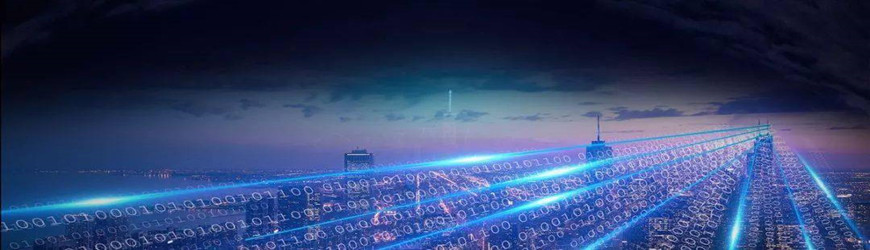
Since the invention of optical fiber decades ago, we have been looking for ways to increase the transmission capacity of the number of optical fiber links. In the early days of optical communication, although we adopted single-wavelength on-off keying (OOK) modulation (turning on and off the laser to indicate "1" and "0"), it can significantly improve the performance of the previous electrical signal transmission, but it also Only a small part of the performance of optical fiber transmission is improved.
Why do you say that? Because the single-wavelength single-mode optical transmission at this time only operates in the low-speed Gbps range, the transmission capacity is defined by the single-wavelength data rate. They do not require light at a specific wavelength or frequency. Optical transceivers with this characteristic are also called "gray light." If you want to transmit a longer distance, it needs to be realized through optical-electric-optical relay.
How can we achieve large-capacity transmission?
The following two major advancements in technologies have played an important role in satisfying large-capacity transmission.
l Multiplexing optical signals of different wavelengths into the same optical fiber for transmission, that is, WDM technology (Wavelength Division Multiplexing),
l Coherent transmission uses a digital signal processor (DSP) to more effectively modulate and detect multiple levels of the phase and amplitude of the laser on the two polarizations, thereby improving spectral efficiency.
Let's take a look at WDM technology first.
In the 1980s, with the invention of the erbium-doped fiber amplifier (EDFA), a major breakthrough in the deployment of optical networks was accelerated. EDFA can enable all wavelengths in the 1550nm low-loss window to be amplified in the optical domain, which means that a single amplifier can be used to amplify multiple wavelengths in the window. Therefore, for long-distance transmission of optical signals, so many expensive relay boards are not needed for electrical regeneration.
At about the same time, the design of lasers and wavelength stabilization technology has matured so that multiple wavelengths can be densely packed in the same 1550nm window. Through EDFA and stable lasers, WDM has also entered the era of Dense Wavelength Division Multiplexing (DWDM) and is still the mainstream technology of optical transmission.
It can be said that the invention of EDFA has greatly promoted the application of DWDM in optical networks, especially the deployment of submarine and long-distance backbone and metropolitan area networks. In 1995, DWDM commercially deployed an 8-wavelength *2.5Gbps WDM network. By 1999, a 40-wavelength *10Gbps WDM system had been successfully deployed.
In order to achieve long-distance transmission, a variety of new technologies have been adopted in the above optical fiber links to overcome various dispersion effects due to the interaction between the laser and the optical fiber medium. For example, in terms of dispersion compensation, dispersion-shifted fiber or passive dispersion compensator modules are mainly used. Of course, this dispersion limitation has been resolved in the 100G/B100G era.
In addition, due to the influence of fiber nonlinearity and the limitation of the ability of directly modulating lasers and direct detection detectors, the OOK modulation method adopted at this time can only support a wavelength division network with a rate of 10Gbps per wavelength, and OOK modulation increases with the rate. The spectral width of the channel will also increase. At the same time, due to the changes in the frequencies of ‘1’ and ‘0’, chirp is inevitable. The existence of chirp broadens the bandwidth of the laser emission spectrum, deteriorates the spectral characteristics of the light source, and limits the transmission rate and distance of the system; suitable for short-distance transmission
Phase modulation was introduced around 2000, and differential phase shift keying (DPSK) and differential quadrature phase shift keying (DQPSK) can be transmitted at a rate of 40Gbps per wavelength. Moreover, these solutions can utilize existing direct detection receiver technology. However, under the same physical conditions, the 40G system is limited by PMD, dispersion and nonlinearity. To achieve the same performance as the existing DWDM 10G, it must have:
l Improve the error correction ability to overcome white noise and reduce the system OSNR requirement by 6dB;
l Advanced coding and modulation technology improves transmission performance in an all-round way,
l Reduce OSNR, PMD, non-linearity, chromatic dispersion and other restrictions.
l New dispersion management technology (such as ADC) to improve dispersion tolerance and reduce non-linearity.
In addition, ITU has played a very important role in the standardization of DWDM and the process of DWDM commercialization. For example, in 2002, the ITU released the frequency grid plan for DWDM transmission, which is the familiar ITU-TG.694.1 Recommendation. This standard is called the grid definition interval is 100GHz, 50GHz and 25GHz, the granularity is 12.5GHz. As shown below.
In the initial stage of DWDM, the frequency spacing is 100GHz and the wavelength spacing is about 0.8nm. This interval is relatively wide, so lasers and passive filters can be manufactured in batches with sufficient margin. Through standardized DWDM design, the operator's network can freely add and drop wavelengths and services on the required nodes.
Of course, at this time, these nodes rely on FOADM, that is, fixed optical add/drop multiplexers to add/drop multiplex. These operations also need to be done manually. For example, when the wavelength changes, you need to manually connect the service board to the M board, and connect the fiber that passes through the wavelength. Later, when tunable lasers or optical filters were invented, we could remotely change the emission wavelength of the business single board through commands on the network management, and there was no need to go to the station to change the pigtail.
With the development of technology, that is, with more stable lasers and optical filtering technologies, DWDM technology can reduce the spacing between adjacent wavelength channels and reduce the frequency spacing from the original 100GHz to 50GHz (50GHz is approximately equal to 0.4nm). Compared with the network using 100GHz spacing, the transmission capacity of the DWDM system is doubled.
Next, we will talk about the role of coherent technology on the capacity of the optical transmission system.
As we mentioned earlier, 10G and 40G incoherent wavelength division networks are more severely affected by fiber dispersion, and dispersion compensation is needed to transmit longer distances. Around 2010, the CMOS integration of high-speed data converters and DSP made coherent optical transmission systems feasible, and coherent optical transmission technology was born.
Coherent technology can compensate the signal dispersion in the electrical domain through algorithms to achieve the ability of 100G and B100G long-distance transmission, but its modulation scheme is much more complicated than OOK, DPSK and DQPSK.
The early coherent scheme used a fixed modulation order and a fixed baud rate for transmission. With the advancement of DSP technology, software programmable modulation order and baud rate become achievable, which is what we now call the SDO function, which provides the ability to use general-purpose hardware to process multiple applications. For example, high-level modulation such as QPSK with 2 bits per symbol, 16QAM with 4 bits per symbol, and 64QAM with 6 bits per symbol can be used to provide greater data transmission capacity.
At the same time, the transmission capacity of the system can also be improved by increasing the rate at which these symbols are transmitted, that is, the baud rate. Of course, modulation and baud rate have a dialectical relationship between transmission capacity and transmission distance. In general, a higher modulation order provides increased capacity at the expense of coverage, while a lower modulation order can transmit farther, but the transmission capacity will be reduced. In order to improve the performance of coherent transmission, technologies such as probability shaping have also been developed to adjust the probability and location of coherent constellation points to optimize coverage and capacity.
At this time, although the coherent technology can be applied to the traditional 100GHz/50GHz channel optical fiber line system. However, as our title says, optical transmission has been seeking greater capacity. In addition to high-order modulation and baud rate, increasing the number of DWDM channels in a single fiber is also one of our efforts. This also puts forward higher requirements for coherent modulation to achieve higher capacity and transmission distance. In other words, we need more flexible frequency and wavelength intervals to support various coherent modulation transmissions, and are no longer limited to 50GHz/100GHz intervals. At the same time, the transmission window is not limited to the current C-band.
Let's talk about the flexible interval first. In order to meet the flexible frequency interval, wavelength selective switch (WSS) technology came into being. Compared with the traditional FOADM fixed add/drop system, the reconfigurable optical add/drop multiplexer (ROADM) composed of WSS.
Such a ROADM system can allow the addition and deletion of specified wavelengths through software control, connecting the fiber once, once and for all. At the same time, WSS technology can also make the frequency interval of our transmission network tunable, that is, a flexible grid DWDM grid that supports FlexGrid instead of a fixed 50GHz or 100GHz interval network, thus allowing different coherent modulations with different spectral widths. Transmission on the same optical fiber.
In response to this demand, ITU-T revised G.694.1 to meet the planning and design of flexible grid spacing networks. At the same time, in 2017, the Optical Interconnection Forum (OIF) also proposed a framework to help develop coherent DWDM transmission with flexibility, including the use of 37.5GHz, 50GHz, 62.5GHz and 75GHz channels.
Solving the problem of flexibility in frequency spacing is to meet the high spectral width requirements of high-rate coherent modulation. But in order to increase the capacity of the entire system, expanding the optical transmission window is also one of the directions we must consider. Especially because of the wide interval brought by the high baud rate, the expansion of the frequency spectrum is particularly important. Therefore, the L-band, which has the smallest transmission loss in the transmission window, has become the first choice for spectrum expansion.
Let's analyze it briefly. For example, we follow the PM-16QAM, 75GHz interval. How to use C-band, the spectrum resource is 4THz. The C-band can only transmit 53 waves, which is actually of little significance. After being expanded to the CE band, the spectrum resource increases by 20% to 4.8THz. If the C++ band is used, it is 6THz. If the C+L band is used, it is 8/12 THz, which is 2 to 3 times the spectrum resources of the C band. However, the limited optical amplification spectrum width and the use of the C+L band require two sets of optical fiber line systems.
Thanks to the aforementioned advancements in multi-person technology, the capacity of optical fiber transmission has doubled many times since the 1980s. The latest transmission solutions have approached the practical limit of optical fiber capacity. However, operators need to plan and design their networks in the most cost-effective manner, taking into account factors such as transmission distance and system capacity, rather than blindly expanding system capacity.